Science with LINC
Taking full advantage of the Fizeau-type optical layout of the LBT interferometer, LINC will offer outstanding research possibilities and enable scientific progress in various interesting astrophysical topics. The main characteristic of LINC and what makes this camera system unique when compared to other interferometric detectors is its unprecedented combination of high angular resolution, high sensitivity for faint sources and large field of view. Especially the latter one is crucial for astronomical studies of extended objects like galaxies and quasar hosts, or for the search for extrasolar planetary systems.
Choosing from a seemingly unlimited spectrum of fascinating astrophysical problems and questions, which will profit from observational data of LINC, the following pages will briefly highlight those research topics, which are intensively investigated at this institute or at our partner institute for the development of LINC, the Max-Planck-Institut für Astronomie in Heidelberg. The pages do not claim to provide a complete summary of every scientific aspect of the respective topic, but should give a short introduction with special respect on the contribution of LINC. For further in-depth information links to the internet pages of other workgroups will be presented where available
Protoplanetary Discs and the Search for Extrasolar Planetary Systems
Circumstellar discs are of fundamental importance for the formation of planets. They not only provide the necessary matter for the planets to be created, but they also act like a shield against the stellar wind, making it therefore possible to form little clumps out of the scattered gas and dust in the first step. The newly formed planet will later on generate a free gap by accretion and resonant scattering and thus changing the structure and dynamics of the disc substancially.
With detailed observations at highest angular resolution and sensitivity it will be possible to search for indications of these changes in the scattered light of the disc. At a wavelength of 1.2 µm LINC will reach an angular resolution of about 20 milliarcseconds. At distances of the nearest molecular clouds (e.g. the Taurus region at 140 parsec) this corresponds to orbital parameters similar to the ones of our earth. The observation of protoplanetary discs might therefore result in the first detection of a planet via its pertubating influence on the structure of the disc. The unique ability of LINC to do real imaging is a great advantage at this point, in contrast to other interferometric cameras.
At increasing age the disc is furthermore expected to flatten, according to the transition from the protostellar to the protoplanetary phase. The detection and monitoring of these structural changes in planetary discs is the objective of a large joint observation program of this institute and the Max-Planck-Institut für Astronomie in Heidelberg.
Currently the search for extrasolar planets is mainly based on two different techniques, either on the observation of Doppler shifted spectral lines from the central object due to the bound motion of the invisible companion, or on photometric changes of the system during occultations. Both techniques are biased towards the detection of heavy jupiter-like objects on tight orbits around the central star. The majority of present detections (about 50 so-called hot-jupiters) shows semi-major axis of less than 0.5 AU, what requires the re-evaluation of current planetary theories.
LINC on the other hand will allow the search for planets using a more classical approach by detecting periodic astrometric changes of the central star because of the gravitational forces of the planet. The very high precision of astrometric studies with LINC opens up for the first time the regime of real jupiter-like planets - planets with a mass comparable to Jupiter's mass moving on orbits of 5 AU around a solar-like star. At normal conditions the achievable relative astrometric precision of LINC will be in the range of 0.1 milliarcseconds. This would already be sufficient to detect the gravitational influence of Jupiter on the motion of our sun from an observing distance of 100 parsec. But at many studied objects it will be possible to improve the astrometric precision even more because of LINC's large field of view, which increases the probability of having multiple reference stars being visible for calibration. In addition the availability of more than one reference star removes any ambiguity, which will be present at measurements of other interferometric cameras: the star which hosts the orbiting planet can clearly be identified by its periodic movement in respect of several neighbouring objects.
Cores of Active Galaxies and Quasars
The high sensitivity of LINC will allow first interferometric observations of the central regions of Active Galaxies and Quasars at infrared wavelengths. After an integration time of 3 hours it will be possible to detect and map a compact source with a signal to noise ratio of 100.
A typical example of these objects is shown in Fig. 1: the circumnuclear dust-disc around the core of NGC 4261 observed at visible wavelengths with the Hubble Space Telescope. Spectroscopic measurements showed that about 40 million solar masses are enclosed with the central 0.1 parsec. The mass to luminosity ratio of about 20 is unusual for stellar populations and gives rise to the assumption that a large fraction of this mass might be present in form of a central black hole.
Using LINC it will be possible to do detailed studies of the core region within 1 or 2 parsec at a high dynamical range. The observed structure of the disc in conjunction with spectroscopic data will allow important insights on the direct accretion of gas and dust onto the core of NGC 4261.
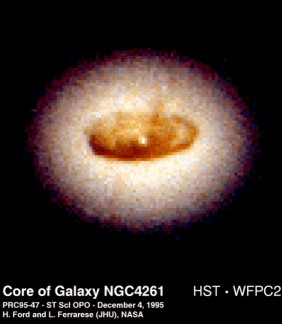
The Black Hole at the Centre of the Milky-Way
Due to its unique combination of high angular resolution and large field of view LINC will be ideally suited for detailed studies of our closest massive black hole, the black hole at the centre of our Milky-Way. Although the galactic centre is located on the southern hemisphere of the sky the LBT will be usable with its full interferometric resolution in east-west direction and unobscured field of view. The galactic centre will be observable for several hours per night what allows at least for a limited aperture synthesis.
The angular resolution of LINC will be about 30 milliarcseconds at a wavelength of 2.0 µm. At a given distance to the galactic centre of approximately 8000 parsec this corresponds to only 250 AU. A comparable angular resolution in combination with the expected sensitivity and field of view is out of reach of any other infrared detector system already in existence or being planned for the near future. LINC will therefore offer unprecedented, outstanding and unique possibilities for detailed astrophysical studies of the direct environment of a massive black hole.
Fig. 1: Proper motion of stars at the central cluster of the Milky-Way (Genzel et al. 2000, MNRAS 317). These pictures have been taken at a wavelength of 2.0 µm with the interferometric speckle camera SHARP 1 at the NTT.
Left image: Motion vectors of hot Helium-stars. For these sources the radial velocity is known as well from infrared spectroscopy. The cross marks the position of the radio source Sgr A* and is in good agreement with the position of an unresolved (radius < 5.8 milliparsec) point mass of 2.6 10E6 solar masses.
Middle image: Similar to the left picture, but with additional motion vectors for all stars brighter than K=13m.
Right image: Motion vectors of stars within a radius of 0.7 arcseconds (i.e. 30 milliparsec or 0.1 lightyears) around Sgr A*. This speckle picture has been taken by Ghez et al. at 2.0 µm at the 10 m Keck I telescope.
When using LINC for observations of the galactic centre the resolution of the pictures will be doubled and the sensitivity for point sources will be increased by several magnitudes, because of the larger collecting area and the adaptive optics system of the LBT.
Fig. 2: Selected keplerian orbits for the central star S2, which is located only a few milliparsec next to the centre. The selected orbits should give an impression of the intrinsic variation of all keplerian orbits, which are consistent with existing observational data from SHARP at the NTT (Eckart et al.) and NIRC at the Keck (Ghez at al.). The orbits of the central stars are bound and show high inclination angles (60° < i < 80°) and eccentricities (0.4 < e < 0.95).
The detailed measurement of stellar orbits in the immediate vicinity of the central mass furthermore allows the precise determination of the gravitational potential and mass distribution at the centre. If the position, motion vector and orbital curvature of an observed star are known altogether it is possible to estimate the amount of enclosed mass out of the parameters of a single object. For the 3 stars located closest to the centre is has so far already been possible to measure their radial orbital acceleration.
Fig. 3: Enclosed mass as a function of the projected and the real distance to Sgr A* as derived from observed radial orbital accelerations. The model calculations demonstrate that the precise knowledge of the central stellar orbits leads to clear statements on the amount, the extent and the distribution of the mass of the central compact object as well as of the inner part of the stellar cluster. The models shown above are based on different mass density distributions of the central mass rho(r) and the stellar cluster n(r) within a radius of 0.4 arcseconds (15 milliparsec) from the centre.
The upper images (a-c) show the lower limits of the enclosed mass as derived from observations without any corrections.
The lower images (d-f) show mass estimates which have been corrected for projection effects.
For the pictures on the left hand side a point mass of 3 10E6 solar masses and constant stellar density have been assumed.
The graphs in the middle of Fig. 3 are based on a point mass of 3 10E6 solar masses as well, but assume a r^(-7/4) gradient of the stellar density.
The graphs on the right hand side show the results of the calculations for an extended central mass distribution rho(r) and a constant stellar number density. The contour lines represent 5%, 10%, 20% and 50% of the maximum density.
With the high angular resolution and sensitivity of LINC it will be possible to observe stars in a distance of only 1 lightday from the central black hole. For these objects relativistic effects like periastron-shift of the major orbital axis or rosette-like orbits due to a mass contribution of the extended central stellar cluster will be observable. Depending on the detailed orbital structure the time of circulation of these stars will only be in the range of a few months. Based on the gradient of the number density of stars towards the position of Sgr A* the detection of such objects in the immediate vicinity of the galactic centre is very likely. Detailed measurements of the orbital parameters will allow definite statements on the amount, the structure and the location of the compact mass in the centre of our Milky-Way.
Fig. 4: The measurements of the central stellar orbits allow the determination of the central mass of the Milky-Way at so far unprecedented small distances to the galactic centre. The two figures presented above show the enclosed mass as derived from observations of the orbital accelerations (Eckart et al. 2001) in comparison to the respective values derived from proper motions (Genzel et al. 2000).
Left graph: Lower limits of the enclosed mass as derived uncorrected from observed orbital curvatures. The results for the two central stars S1 and S2 are shown as observed with SHARP at the NTT (Eckart et al. 2001) and NIRC at the Keck (Ghez et al. 2000).
Right graph: The presented mass estimations are statistically corrected for projection effects of S1 and S" within a central volume from 0.3 arcseconds (11 milliparsec) to 0.5 arcseconds (19 milliparsec) in radii from the galactic centre (area shaded in light grey).
Based on currect interpretations the luminosity of Sgr A* results from advective accretion of matter onto a massive black hole. Recent observations at near infrared wavelengths might indicate a possible identification of the radio-source Sgr A* with a variable infrared source (Genzel, Eckart, Ott et al.). This would be the first detection of Sgr A* outside the radio regime and allow further measurements, which will contribute decisive to further studies of the physical properties of this source.
Further in-depth information on the studies of the massive black hole at the centre of the Milky-Way can be found on the web pages of the galactic centre workgroup at this institute: http://www.astro.uni-koeln.de/gc/
Supernova Cosmology
Studying supernovae at moderate redshifts has changed our view of the large structure of the universe dramatically within the last years. The basic point of the observations is the fact that supernovae of type Ia show a known relationship between the evolution of their lightcurves in time and their intrinsic luminosities. With individual measurements of the lightcurve and the spectroscopic redshift it is therefore possible to obtain a relation between the luminosity-distance and the redshift of the supernova dependig on omega_m and omega_lambda.
Unfortunately only supernovae at moderate redshifts are accessible using present telescopes and the analysis of these objects only leads to a limitation of a combination of omega_m and omega_lambda and not of the individual values itselves. However, with observations of supernovae type Ia at redshifts of z=2.5 it would be possible to break up this degeneration of parameters.
LINC will provide sufficient sensitivity to study those objects in great detail. Assuming an observing programm of approximately 25 nights it will be possible to determine the density parameter omega_m with a remaining error of measurement of about 5%.
Galaxy Formation
The best way to study the formation of galaxies is to use the large collecting area of a modern telescope and look back in time to the era of galaxy formation. Based on current theories the first fragments of galaxies are very small and faint objects. Nevertheless, due to the limited sensitivity of existing astronomical detectors, the present studies are biased towards unusually luminous and massive galaxies, as well as towards galaxies undergoing a period of increased star formation.
With LINC it will be possible to compile a deep multi-colour near-infrared survey within 20 observing nights, which covers a volume of about 10E5 megaparsec³ and in which fragments of galaxies with only one thousandth of the mass of the Milky-Way can be detected. Up to the commissioning of the Next Generation Space Telescope no other camera system will reach this combination of high sensitivity, wide spacial coverage and large number of expected objects to be detected.
Spatially Resolved Imaging of Extragalactic Stellar Populations
The ability to study individual stars in galaxies beyond of about 5 megaparsec is limited by present camera systems. The evaluation of stellar constitution and evolution of these galaxies has therefore to be based on the spectral distribution of energy of the unresolved objects. In particular there is not a single elliptical galaxy close enough to be completely resolved in individual stars.
With LINC it will be possible for the first time to resolve stellar populations up to distances of 20 megaparsec. Using broad and narrow-band spectral filters it will furthermore be possible to determine the age and metallicity of these stars.
Compared to the low number of 4 of these objects, which have been studied so far in more or less detail, LINC will be able to access individually resolved stars in about 100 luminous galaxies for detailed investigations. At larger distances the high sensitivity and angular resolution of LINC can be used to observe luminosity fluctuations in marginally resolved stellar populations. These measurements give a clear indication of the object's distance (Tonry & Schneider 1988). As the maximum observable distance of such studies scales with the square root of the extent of the point spread function, LINC will offer a 1000 times larger volume for inspection than any camera system existing so far.
Energy Balance at the Early Stages of Stars
Stellar winds are of great importance for the support and the regulation of star formation because of their interactions with circumstellar discs and the surrounding interstellar medium. Unfortunately respective studies have so far been severely limited by the small angular extent of the regions of interaction and the high absorption by dust particles.
LINC, however, will offer unprecedented opportunities for detailed studies of these processes. At the closest star forming regions the angular resolution of LINC will correspond to a transversal extent of less than 1 AU. In respect of other interferometric detectors the unique ability of LINC to deliver real images is a great advantage for the data reduction of the complex interacting regions.
An observing program for circumstellar emission in conjunction with high resolution spectroscopy can furthermore monitor the complete 3-dimensional motion of the gas in close vicinity of the star. For a shock wave running at 25 km/s in Taurus for example it would be possible to detect its significant movement within a single observing run of one week.
Double and Multi Stellar Systems
The majority of main sequence stars in our galaxy is located in double or multi stellar systems (Duquennoy & Mayor 1991). The distribution of the orbital semi-major axis of double stars shows a maximum at about 50 AU, what corresponds to approximately 0.3 arcseconds at our nearest star forming regions.
At infrared wavelengths LINC will be able to penetrate the obscuring dust clouds and resolve individual stars in young binary systems down to an angular separation of 0.02 arcseconds. Even the moderate spectral resolution of a grism (which is included in a later on improved version of LINC) will be sufficient for the spectral classification of the individual objects and therefore for the proper determination of their placement at the Hertzsprung-Russel-Diagram and their evolutionary state (Meyer 1996).
The large field of view of LINC will furthermore allow to do astrometry at an extraordinary high level of precision and to determine the orbital parameters for a large number of binary systems. The derived dynamical masses can be used to calibrate the mass-luminosity relation and the pre-main sequence of the Hertzsprung-Russel-Diagram.
Detailed Imaging of Planetary Surfaces and Atmospheres in our Solar System
For astronomical targets within our solar system the large field of view, the high angular resolution and the high sensitivity of LINC will allow detailed studies at very high quality, being comparable to satellite based detectors. The high level of detectable details could even lead to occasional problems, because the characteristic time span of atmospherical changes at the observed object could sometimes be shorter than the necessary observing time to cover most parallactic angles, which are related to the daily rotation of the earth.
Imaging of the atmosphere and surface of Titan, the largest moon of Saturn, will be a very important observation program. As Titan is the only other object in the solar system with oceans and precipitation it is the designated target of many studies already going on or being started in the near future.
Some planetary scientists assume that the atmosphere of Titan resembles terrestrial conditions before the evolution of life and the atmospherical Oxygen being generated by these lifeforms.
The penetration of the thick cloud layer of Titan in the infrared will furthermore allow images of the surface of this moon with so far unique clarity and spatial resolution.
At the spectral K-band the angular resolution of LINC will be about 20 milliarcseconds, what corresponds to approximately 130 km at the distance of Saturn. The diameter of Titan will therefore cover about 40 pixels on the detector array. For comparison the respective resolution of the Hubble Space Telescope is about ten times more coarse and the diameter of Titan would cover only 4 pixels.
Observations of Titan with LINC could furthermore become very newsworthy: The Cassini satellite is scheduled to arrive at Titan at about the same time as the commissioning of LINC at the Large Binocular Telescope.